What can chemists learn from nature?
Tuesday 18th Apr 2017, 11.22am
Nature is full of chemicals – flavours, fragrances, medicines. Living systems have been making these useful chemicals for billions of years, but usually only tiny quantities, because that’s all they need! In this animation we find out how chemists are learning from nature to create these chemicals in much larger quantities, to satisfy our industrial needs.
Enzymes are the catalysts inside all living cells. They are responsible for speeding up chemical reactions, turning one chemical (the starter chemical) into another chemical (the product).
This work focuses on very tricky reactions, where 2 hydrogen atoms are added across a double bond. When this happens, two products are often made – one is ‘right handed’ and one is ‘left handed’. Although these two products are chemically very similar, they can be very different biologically. This is because enzymes are very specific, and can recognise the correct starter chemicals and products.
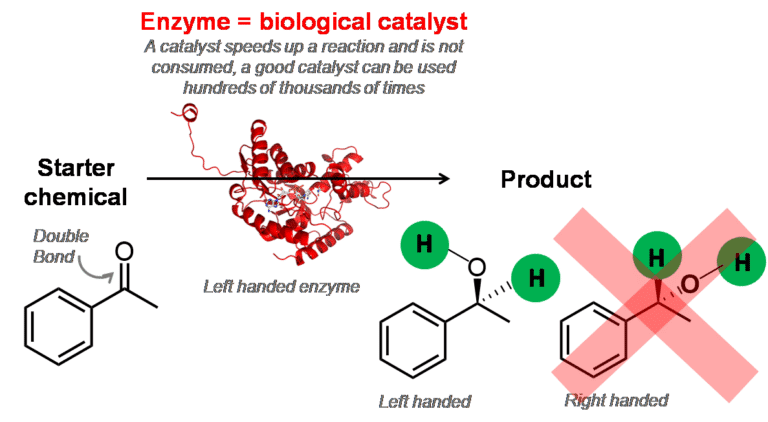
Precision is key
It’s very important that these reactions happen perfectly, because if the wrong chemical is made, this can cause big problems inside cells. For example, enzymes carry out reactions inside our bodies; if they make mistakes here, there is a chance the resulting chemicals will affect our health. Fortunately, enzymes have evolved over millions of years, and usually operate with perfect precision.
Enzymes also allow cells to carry out reactions that usually require lots of energy, under very mild conditions. To do the same reactions in the lab, we might need to use very high temperatures or very high pressures. This is not very sustainable and there are often high costs, both economically (they are expensive!) and environmentally (lots of energy required = bad for the environment!).
For all these reasons, many scientists are now using biological systems, including enzymes, to do chemistry in laboratories. Our work focuses on cases where a particular enzyme is taken out of cells and used for a desired reaction.
Time for a recharge
Many enzymes work perfectly when taken out of cells, which may be surprising! However, lots of the most useful enzymes only work in the presence of a special helper molecule, NADH.
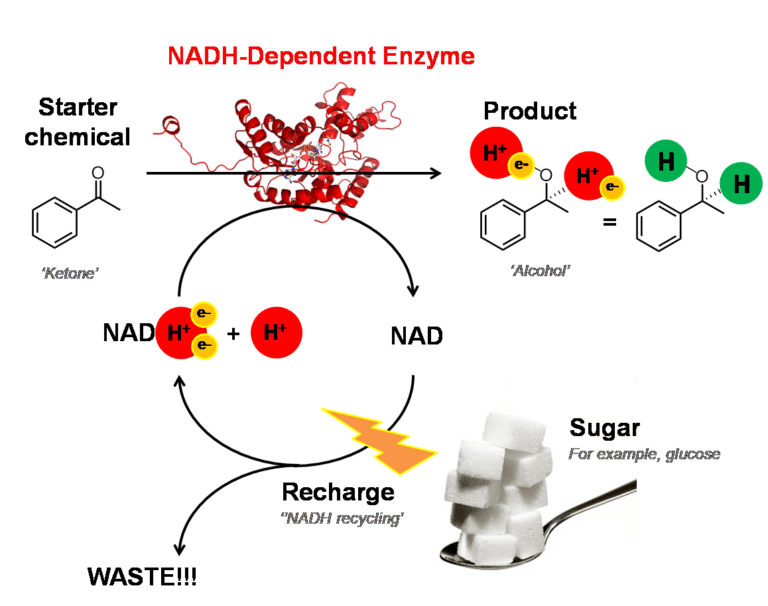
This NADH supplies electrons for a reaction (like a power pack), but once the starter chemical has been turned into the product, the helper molecule needs recharging. There is infrastructure inside cells to recharge the NADH but when we use isolated enzymes we have to find a second biological machine, another enzyme, to recharge the NADH. This ‘NADH recharging machine’ needs to be powered by chemical energy. In the methods presently used in industry, this chemical energy is usually supplied as glucose (sugar). However, this has significant limitations because the glucose is a carbon intensive energy source and makes a lot of chemical waste products.
We are increasingly familiar with hydrogen gas (H2) as a clean energy source. Chemists started using hydrogen gas as a clean energy source for reactions a long time ago, in fact, many chemists have won Nobel prizes for it! This is because many reactions actually require addition of H2 (two hydrogen atoms) across a double bond – using H2 as the ‘energy’ source means that the reaction is highly efficient and that no waste is generated. Although very elegant, these chemical methods rely on toxic, expensive, metal catalysts, and do not meet the same selectivity that enzymes do.
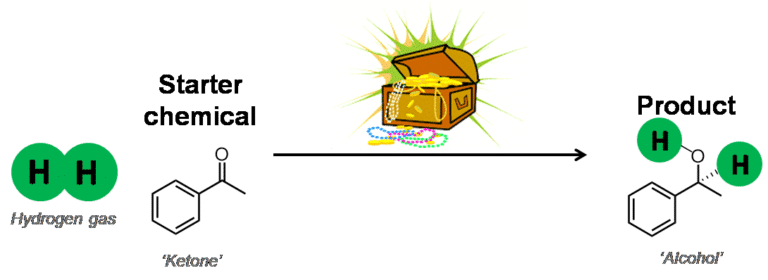
The best of both worlds
Inspired by both the chemical and the biological methods, Holly and Kylie set to work developing a system that would bring together the advantages of both! They have developed a robust system that enables the use of hydrogen gas to drive the recharging of NADH to power enzyme reactions. Specialised enzymes that contain metal sites allow the enzymes to be ‘plugged’ onto an electronically conducting carbon bead (to form an electric circuit) and use hydrogen gas as a clean and efficient electron source to drive recharging of NADH. Any NADH-dependent enzyme can also be ‘plugged in’ to carry out highly selective chemical reactions without generating any waste products.
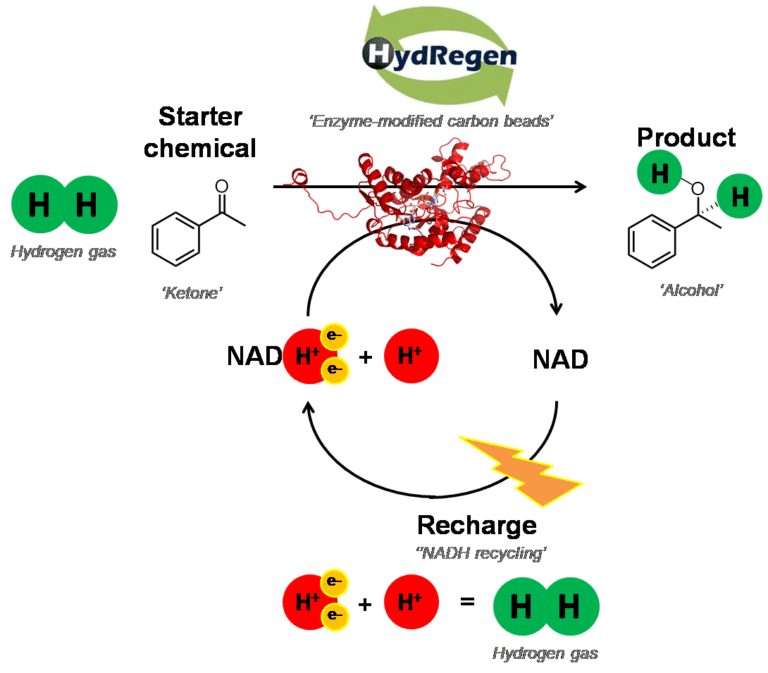
This technology, HydRegen, brings together the near perfect selectivity of biology and the elegance and efficiency of chemistry. In the future, this may allow more chemicals to be made using enzymes and help us make chemicals more cleanly.
Holly and Kylie have developed a robust system that enables the use of hydrogen gas to drive the recharging of NADH to power enzyme reactions
What kind of chemicals can we make?
At the moment, we are still testing this out on simple reactions. But, NADH dependent enzymes are used for all kinds of useful reactions. Oxford Biotrans (listen to our podcast about how you can turn a grapefruit into an orange here) use NADH-dependent enzymes to turn oranges into grapefruits!! Lots of companies use them for really tricky chemical steps in the production of medicines. In the future, we hope that even more chemicals we be made using enzymes and that this will be made easier because of the HydRegen system.
I’m still intrigued! How does HydRegen actually work?!
The HydRegen systems uses very specialised enzymes, immobilised on a carbon bead. The first enzyme, hydrogenase (green) can rip hydrogen gas (H2) apart forming protons and electrons. The enzyme has a ‘wire’ of metal clusters which means the electrons can be transported into the carbon bead. The next enzyme, NAD+ reductase (blue) can collect these electrons, along with a proton from solution and use them to recharge NAD+ to make NADH. Then the NADH-dependent enzyme can use the NADH for a desirable reaction! This NADH can be used time and again, and so can the enzyme-modified particles. This should help to significantly reduce the cost of biocatalysis in the future.
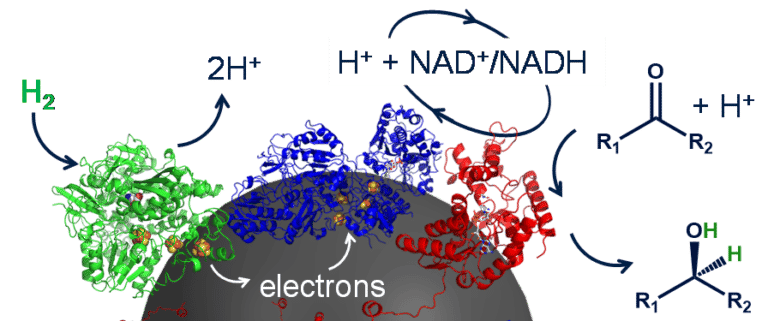
Contact us to find out about the following teaching resources:
KS3: Flavour Saver
KS4: Green Vanilla
KS5: Organic Pathways
KS5: Biology to Technology
Click here to find out more about the Kylie Vincent’s Group here
Click here to watch this video on HydRegen is making cofactor-dependent catalysis cleaner
Click here to read this science blog article on the research
Click here to listen to our podcast with Kylie Vincent about her research
Click here to find out more about Holly Reeve here